Overview and Description
Based on information available from the National Center for Health Statistics, it is estimated that in the United States there are 50,000 new amputations every year. Data from the National Health Interview on Disability (NHIS-D) reports one in 200 Americans is living with either upper or lower limb loss.1 Although lower limb loss is more prevalent (80%) than upper limb (10%) or multiple limbs (10%), upper limb (UL) loss has its own unique challenges and concerns. Of the 41,000 persons with major UL loss in the U.S reported in 2005, 62% were trauma related.2 Trauma related amputations usually occur as a result of motor vehicle, military conflict, industrial or farming accidents and may account for up to 30% of new major limb amputations. Traumatic amputations occur in the younger, active, and economically productive population. Sixty percent of persons with arm amputations are between the ages of 21 and 65 years, and 10% are younger than 21 years. Because of the higher risk of work-related accidents in men, there is a higher number of trauma-related amputations for this gender and, overall, a higher incidence of upper limb amputation. The National Center for Medical Rehabilitation Research, within the National Institutes of Health, in partnership with the Department of Defense has funded the creation of the Limb Loss and Preservation Registry (LLPR) with the goal to collect reliable data to make informed analytical decisions.3
Upper limb amputations are demarcated at the wrist joint; distal to the wrist are considered minor amputations, whereas proximal to the wrist are major limb amputations. Amputations levels are forequarter (interscapulothoracic), shoulder, transhumeral, elbow disarticulation, transradial, wrist disarticulation, transcarpal, transmetacarpal, and transphalangeal level. The most frequent major upper limb amputation is at the transradial level, which accounts for over half of all major arm amputations.4,5
Prosthetic prescription, fitting and training on use is one of the primary treatments of functional impairment resulting from amputation in an effort to restore function and improve quality of life.
Early prosthetic fitting and training with the device has been noted as a major factor for prosthetic acceptance.6 Therefore, the amputation rehabilitation program should ideally begin as early as possible and in elective procedures, prior to the amputation.
The complicated multi joint movements and functions of the upper extremity have yet to be replicated with prostheses and patients that utilize prosthetics often cite limited dexterity and lack of sensory feedback as primary reasons for abandoning the prosthesis.7
The need for a multidisciplinary team approach is acknowledged in the first evidence-based clinical practice guidelines for the rehabilitation of persons with upper-limb amputation, released in 2014.8
Relevance to Clinical Practice
Prosthetic prescription
Factors to consider when selecting and prescribing prosthetic components are level of amputation, residual limb sensation, proximal joint range of motion, strength, soft tissues condition, cognition, vocation, hobbies, importance of cosmesis, financial resources available, and environment and weather. Collaboration between the patient and physiatrist-led rehabilitation team should be in place at the time of prescription. Major components of upper limb prostheses include the terminal device (TD), interposing joints, socket, suspension, and control systems. Typically, there are three general classes of upper extremity prostheses: passive function (cosmetic), body-powered and myoeletric.9,10 There are also hybrid type prostheses available. When considering the type of device and the type and amount of prosthetic training prescribed, a comprehensive clinical assessment by a trained multidisciplinary team can help in determining the appropriateness and readiness for use of an upper limb prosthesis and help guide the prescription of the device or devices and a training program to meet the individual’s needs and goals.11
Control system
Prostheses can be controlled using body-power, external power, or hybrid control systems. Body-powered systems use body movements to control a TD and/or elbow. Advantages of the body powered systems include durability, reduced weight and cost, compared with other systems. They also offer speed of action and some proprioceptive feedback to the user through the harness.
Externally battery-powered systems may use myoelectric signals or switches for control. Electric switches can be activated by residual limb movements within the socket or by other body parts. Myoelectric systems use electromyographic (EMG) signals generated during muscle contractions. They can be used for digital (on/off) or proportional control. With proportional control, the velocity of motor action is proportional to the EMG signal amplitude allowing for variable speed and force of the device. Externally powered systems may reduce the need for harnessing and require less joint movement for activation, but they are heavier, more costly and may require more maintenance than body-powered devices. Figure #1
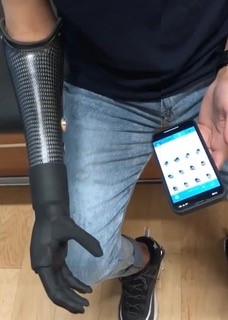
Figure # 1 Myoelectronic transradial level prosthetic device with suction socket, flexible interface and multiarticulate hand. An APP on the phone is used to control various grips available
Hybrid control systems use a combination of body and external power control components in an effort to balance weight, cost, and cosmesis and accommodate different anatomic levels or abilities.7,11 The current literature is insufficient to categorically determine the functional superiority of myoelectric versus body powered prostheses. Body powered prostheses are generally more tolerant to wet or dirty environments and may require shorter training periods. Myoelectric devices can provide better cosmesis, are more accepted for light intensity or repetitive work and influence phantom limb.11 Prosthetic users may have access to multiple devices on a daily basis. One survey of 50 service members from the Operation Enduring Freedom / Operation Iraqi Freedom with unilateral amputation found that within 1 year of their amputation many used more than one type of device that was available to them.12
Terminal device
The current level of prosthetic technology is far from achieving replacement of the versatility, high dexterity and sensation of the human hand, although prosthetic technology is continually advancing. Prosthetic TDs include passive, body-powered, and externally powered hooks and hands. Passive hand TDs are used largely for cosmetic restoration with some stabilizing functions.
Prosthetic hands provide 3-jaw chuck pinch, newer multiarticulate hands have more grip options and hooks provide the equivalent of lateral or tip pinch. Body-powered control allows for voluntary opening (VO) or voluntary closing (VC) of the TD, but not both. VO devices are maintained in the closed position by rubber bands or springs and open when tension is applied through a cable connected to a harness. VC devices are maintained in the open position and close when tension is applied through a cable connected to a harness. VC TDs are capable of applying more force, but VO TDs are more practical because tension does not need to be maintained when holding objects.13
Externally powered TDs can have digital or proportional control and can open or close as desired and offer the advantage of higher grip force.14
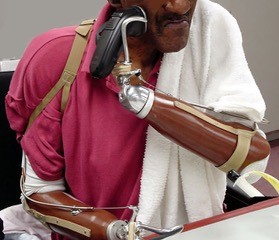
Figure #2 Prosthetic wrist that allows rotation and flexion
The lightest and simplest prosthetic wrist is a friction control unit, which permits passive pronosupination of the TD but can inadvertently rotate when lifting heavier objects (e.g., a plate of food). A locking, quick disconnect wrist allows locking the device in the desired pronation/supination position and offers potential rapid interchange between different TDs. Spring-assisted wrist flexion is helpful to bilateral amputees to permit midline reach for activities of daily living (ADLs) as seen in figure #2
Externally powered wrist units are prescribed more frequently for bilateral transhumeral or higher levels of amputation. Some wrists are capable of 360˚ rotation, which may be used for key turning or operating a screwdriver.7,14
New technologies for myoelectric prostheses
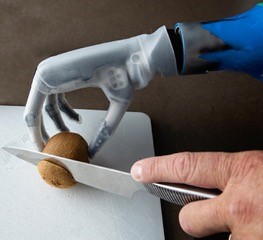
Figure # 3 Multiarticulated myoelectric proportional control hand with wrist rotation and flexion.
Prosthetic elbow
Body-powered elbows can have spring-assisted flexion. External mounted elbows are indicated for elbow disarticulations in attempts to maintain better arm length proportions. Passive and body-powered elbows have a locking mechanism that can be activated with the contralateral hand, chin, or ipsilateral shoulder motion. When used with a body-powered TD, the elbow must be locked in order to transfer the force from the harness to operate the TD.
Externally powered elbows can be controlled with a switch or myoelectric control alternating with the TD. Internal and external rotation can be provided with a rotating turn table, which enables midline reach.
Prosthetic shoulder
Most shoulder joints allow passive abduction and flexion through a bulkhead or universal joints with the desired position maintained by friction, chin control, or electric lock. However, there is increased risk of nonuse because of a combination of weight, diminished overall control across multiple joints, and increased effort with shoulder or forequarter amputations. Powered shoulder with electronic controls is available for individuals with high level transhumeral amputations.14
Prosthetic socket
Most upper limb prosthetic sockets are double walled and composed of carbon graphite or plastic resin lamination materials to which the necessary prosthetic components are attached. The inner socket is fabricated from a cast of the patient’s residual limb and can also be constructed of a flexible thermoplastic or a plastic laminate. Windows can be cut in the outer socket to allow for flexible inner socket expansion. Although more costly to fabricate, the frame design may allow for periodic inner socket replacement to accommodate residual limb volumetric changes.7,8
Suspension system
The major types of suspension are harness, anatomical, friction, and suction suspension. Except for suction suspension or myoelectric control that requires direct skin to socket contact, socks can be used as an interface between the residual limb and socket to accommodate for physiologic volume changes that occur throughout the day and protect the skin and facilitate hygiene.
The harness can provide suspension of the prosthetic device to the body while also serving to providing body-powered control. A figure 8 harness is commonly used for transradial and transhumeral amputees. A harness loops around the contralateral axilla to anchor the suspension and control the cable. A chest strap is an alternative for those who find the axillary pressure uncomfortable. A shoulder saddle with a chest strap can be used in more proximal amputations and for those who do heavier lifting.
A cable connected to the harness allows transmission of body power for prosthetic control. A cable used to activate a single prosthetic component is called a single-control cable or Bowden cable system. A dual-control cable system uses one cable to control two prosthetic functions (e.g., flexion of the elbow, activation of the TD when the elbow is locked). This is accomplished by passing a single cable through two separate sections of cable housings (fair lead cable system). In transradial and transhumeral amputations, biscapular abduction and/or humeral flexion control elbow flexion and/or the TD. To lock or unlock the elbow, shoulder depression, humeral abduction, and humeral extension are performed simultaneously. Patients with shoulder disarticulation perform biscapular abduction to control the elbow and TD with scapular elevation to lock the elbow.13,14
Anatomic (self-suspension) systems use bony prominences for suspension encasing the medial and lateral epicondyles. The supracondylar (Müenster or Northwestern) suspension can be used with transradial amputations and some wrist disarticulations with some loss in end of range of motion. A figure 9 harness is incorporated for TD control only. This suspension works well with externally powered prostheses as well and is less restricting when flexible materials and frame cutouts are incorporated in the design as discussed earlier.
Silicone sleeves provide suspension by creating negative atmospheric pressure and an adhesive bond to the skin. The sleeve also protects the skin by reducing shear forces and providing some cushioning. Therefore, it is useful when the skin is delicate because of scars or injury. It is easily donned with one hand and allows for some residual limb volume change accommodation. There is often a distal attachment pin that interfaces with a shuttle lock mechanism built into the socket. After spraying the external surface with a lubricating fluid, the patient rolls the sleeve directly over the skin. Once in place, socks can be applied to adjust fit. Disadvantages to this suspension are excessive perspiration, the need for diligent cleaning and skin irritation that can occur limiting their use in warm or humid weather.
Suction suspension is preferred for transhumeral amputees with externally controlled devices. The patient dons the socket using a pull sock or lubricant fluid with a 1-way valve to allow for expulsion of air to create negative pressure. For this system to work well, it requires intimate fit between the residual limb and socket to create a tight seal; therefore, the limb volume should be stable with minimal soft tissue surface irregularities.
Expected functional outcomes and realistic goals for most unilateral transradial and transhumeral amputees are independence in all ADLs, household activities, and driving. Some limitations regarding work and household chores may be necessary, especially when dealing with the handling of delicate, heavy, or voluminous objects. Bilateral amputees should be able to perform most ADLs and household activities after assisted donning of the prosthesis. They may also drive with a spin ring and perform some sedentary work with environmental modifications.
Cutting Edge/Unique Concepts/Emerging Issues
The intended goal for upper extremity limb amputation rehabilitation and prosthetics and the advancement in technologies in the fild is to recreate the complex multi dexterous functions of the human upper limb. Recent advances include prosthetic hands with independently controlled finger movements, targeted muscle reinnervation to expand control options, neural prosthesis interfaces and the incorporation of tactile feedback.15-18
As currently available, fall short of restoring the sense of touch or proprioception of the amputated limb and they are perceived as heavy by the users. The lack of sensation makes control of grip force problematic, as prosthesis users cannot tell how hard they are gripping objects; thus, they are susceptible to dropping items because of too little or improper grip. Research is currently investigating sensory feedback to prosthesis users an area that needs further development.19
Osseointegration is an evolving surgical technique to allow the direct attachment of an external prosthesis to the skeleton in select patients who can’t tolerate conventional sockets, thereby eliminating issues such as discomfort, skin breakdown, and variable fit. Multiple percutaneous implant systems exist for clinical use, each attempting to create a stable bone-implant interface while avoiding complications such as infection and loosening.17,18
Improvement in power supply, sensors, newer materials and reduction in the size and weight of components should allow for further improvement in prosthetic devices and outcomes.7,18-19
Implementation of pattern recognition algorithms has allowed the user to correlate a specific EMG pattern with a corresponding preprogrammed upper limb action rather than simply “hand open” or “hand closed” models. This advancement allows for simultaneous control of multiple degrees of freedom, more intuitive and adaptable control of the prosthesis and elimination of the need for action mode switching.20
Myoelectric sensors (bions) can be implanted beneath the skin to improve the prosthetic function and provision of biomimetic bidirectional volitional motor control of, and. Sensory / proprioceptive feedback from external prosthetic devices. 21,22
3D Printing in upper limb prosthetics
3D printing developed in the last 3 decades has evolved with significant advancement in the printing of upper extremity prostheses. There have been many publications in the scientific community about research regarding the field of 3D-printed upper limb prostheses. Largely the development of 3D printed upper extremity prosthetics came about due to a necessity to develop more affordable prosthesis particularly to address skeletal growth in children and the provision of personalized socket, shape, size and color at a lower cost.23 The global community e-NABLE has grown into a worldwide interdisciplinary movement.24
Virtual reality and advanced rehabilitation
Interdisciplinary rehabilitation teams are exploring how to implement virtual reality programs to optimize prosthesis design and training to more efficiently train upper extremity amputees using a virtual avatar.26 Game-based training techniques can improve myoelectric prosthesis control and could potentially allow training outside the clinic.27
Finger and partial hand amputation rehabilitation
As described in the overview, the great majority of upper limb amputations affect the fingers. Traditionally cosmetic fingers or gloves have been the primary intervention with limited functional impact. A new solution for this very large group of patients that was not available in the past is the application of functional finger prosthesis. These devices use clever biomechanical design to allow finger flexion and gripping of objects with custom simple design and high functional outcomes.29
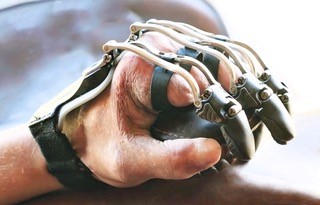
Figure #4 Partial finger prosthesis with functional grasp
Gaps in Knowledge/Evidence Base
A gap exists in knowledge about the impact of upper-limb loss, prosthesis use and amputation rehabilitation on activity and participation, specifically work participation. This approach may result in the development of guideline for prescription of prosthetics and training.30 Except for prosthetic centers of excellence in the Veterans Health Administration, data is limited on the location, outcomes and level of expertise of self-described centers of excellence for the care of persons with upper limb amputation across the US. 31 Development of resource utilization rational, cost-benefit analyses and coverage for devices and related services would be a great step forward.32
References
- Centers for Disease Control and Prevention (CDC. “Hospital discharge rates for nontraumatic lower extremity amputation by diabetes status–United States, 1997.” MMWR. Morbidity and mortality weekly report 50.43 (2001): 954.
- Ziegler-Graham, Kathryn, et al. “Estimating the prevalence of limb loss in the United States: 2005 to 2050.” Archives of physical medicine and rehabilitation 89.3 (2008): 422-429.
- https://www.llpregistry.org
- Tennent DJ, Wenke JC, Rivera JC, Krueger CA. Characterisation and outcomes of upper extremity amputations. Injury 2014; 45:965.
- Esquenazi A, Flack M, Garcia D and Lerman D. Prosthesis for the Upper Limb in Chapman’s Comprehensive Orthopaedic Surgery (Chapman and James edt.) Chapter 269, 2019.
- Malone J, Fleming LL, Roberson J, et al. Immediate, early, and late postsurgical management of upper-limb amputation.J Rehabil Res Dev.1984;21:33-41.
- Esquenazi A. Amputation rehabilitation and prosthetic restoration. From surgery to community reintegration.Disabil Rehabil.2004;26:831-836.
- Management of Upper Extremity Amputation Rehabilitation Working Group. VA/DoD clinical practice guideline for the management of upper extremity amputation rehabilitation. Washington, DC: VA, Department of Defense (DoD); 2014.
- Pierrie SN, Gaston RG, Loeffler BJ. Current Concepts in Upper-Extremity Amputation. J Hand Surg Am. 2018 Jul;43(7):657-667. doi: 10.1016/j.jhsa.2018.03.053. Epub 2018 Jun 2. PMID: 29871787.
- National Academies of Sciences, Engineering, and Medicine; Health and Medicine Division; Board on Health Care Services; Committee on the Use of Selected Assistive Products and Technologies in Eliminating or Reducing the Effects of Impairments; Flaubert JL, Spicer CM, Jette AM, editors. The Promise of Assistive Technology to Enhance Activity and Work Participation. Washington (DC): National Academies Press (US); 2017 May 9. 4, Upper-Extremity Prostheses.
- Carey SL, Lura DJ, Highsmith MJ. Differences in myoelectric and body-powered upper-limb prostheses: Systematic literature review. Journal of Rehabilitation Research & Development. 2015;52(3):247–262.
- McFarland LV, Winkler S, Jones MW, Heinemann AW, Reiber GE, Esquenazi A. Unilateral upper limb loss: Satisfaction and prosthetic device use in service members from Vietnam and OIF/OEF conflicts. Journal of Rehabilitation Research & Development. 2010;47(4):275–298.
- Barton JE, Sorkin JD. Design and evaluation of prosthetic shoulder controller. J Rehabil Res Dev. 2014;51(5):711-726. doi:10.1682/JRRD.2013.05.0120
- Behrend C, Reizner W, Marchessault J, Hammert W. Update on advances in upper extremity prosthetics.J Hand Surg Am.2011;36:1711-1717.
- O’Doherty J, Lebedev M, Ifft P, et al. Active tactile exploration using a brain-machine-brain interface.Nature. 2011;479:228-231.
- Kuiken TA, Dumanian GA, Lipschutz RD, Miller LA, Stubblefield KA. The use of targeted muscle reinnervation for improved myoelectric prosthesis control in a bilateral shoulder disarticulation amputee. Prosthetics Orthot Int. 2004;28:245–253
- Bates TJ, Fergason JR, Pierrie SN. Technological Advances in Prosthesis Design and Rehabilitation Following Upper Extremity Limb Loss. Curr Rev Musculoskelet Med. 2020;13(4):485-493. doi:10.1007/s12178-020-09656-6
- Jönsson S, Caine-Winterberger K, Brånemark R. Osseointegration amputation prostheses on the upper limbs: methods, prosthetics and rehabilitation. Prosthet Orthot Int. 2011 Jun;35(2):190-200. doi: 10.1177/0309364611409003. PMID: 21697201.
- P. P. Vu et al., “Closed-Loop Continuous Hand Control via Chronic Recording of Regenerative Peripheral Nerve Interfaces,” in IEEE Transactions on Neural Systems and Rehabilitation Engineering, vol. 26, no. 2, pp. 515-526, Feb. 2018, doi: 10.1109/TNSRE.2017.2772961.
- Sensinger Jonathon W and Dosen Strahinja A Review of Sensory Feedback in Upper-Limb Prostheses From the Perspective of Human Motor Control. Front. Neurosci., 23 June 2020 Sec. Neural Technology Volume 14 – 2020 | https://doi.org/10.3389/fnins.2020.00345
- Zaid, Musa B. MD; O’Donnell, Richard J. MD; Potter, Benjamin K. MD; Forsberg, Jonathan A. MD, PhD. Orthopaedic Osseointegration: State of the Art. Journal of the American Academy of Orthopaedic Surgeons 27(22):p e977-e985, November 15, 2019. | DOI: 10.5435/JAAOS-D-19-00016
- Purushothaman Geethanjali (2016) Myoelectric control of prosthetic hands: state-of-the-art review, Medical Devices: Evidence and Research, 9:, 247-255, DOI: 10.2147/MDER.S91102
- elle ten Kate, Gerwin Smit & Paul Breedveld (2017) 3D-printed upper limb prostheses: a review, Disability and Rehabilitation: Assistive Technology, 12:3, 300-314, DOI: 10.1080/17483107.2016.1253117
- Enabling The Future [Online]. [cited 2015 Jun 19]. Available from: http://enablingthefuture.org/
- Robbie Brack, Emeka H. Amalu, A review of technology, materials and R&D challenges of upper limb prosthesis for improved user suitability, Journal of Orthopaedics, Volume 23, 2021, Pages 88-96, ISSN 0972-978X, https://doi.org/10.1016/j.jor.2020.12.009.
- Boschmann, A., Neuhaus, D., Vogt, S. et al. Immersive augmented reality system for the training of pattern classification control with a myoelectric prosthesis. J NeuroEngineering Rehabil 18, 25 (2021). https://doi.org/10.1186/s12984-021-00822-6
- Melero M, Hou A, Cheng E, Tayade A, Lee SC, Unberath M, Navab N. Upbeat: augmented reality-guided dancing for prosthetic rehabilitation of upper limb amputees. J Healthcare Eng. 2019;2019:1–9.
- Schiefer M, Tan D, Sidek SM, Tyler DJ. Sensory feedback by peripheral nerve stimulation improves task performance in individuals with upper limb loss using a myoelectric prosthesis. Journal of Neural Engineering. 2016;13(1):016001
- Graham EM, Hendrycks R, Baschuk CM, Atkins DJ, Keizer L, Duncan CC, Mendenhall SD. Restoring Form and Function to the Partial Hand Amputee: Prosthetic Options from the Fingertip to the Palm. Hand Clin. 2021 Feb;37(1):167-187. doi: 10.1016/j.hcl.2020.09.013. PMID: 33198915.
- Darter BJ, Hawley CE, Armstrong AJ, Avellone L, Wehman P. Factors Influencing Functional Outcomes and Return-to-Work After Amputation: A Review of the Literature. J Occup Rehabil. 2018;28(4):656-665. doi:10.1007/s10926-018-9757-y
- Etter K, Borgia M, Resnik L. Prescription and repair rates of prosthetic limbs in the VA healthcare system: Implications for national prosthetic parity. Disability and Rehabilitation: Assistive Technology. 2014;10(6):1–8
- Stark G. Competency, risk, and acceptance of upper limb prosthetic technology. Washington, DC: 2016. [May 16]. Presentation to the Committee on the Use of Selected Assistive Products and Technologies in Eliminating or Reducing the Effects of Impairments.
Original Version of the Topic
Alberto Esquenazi, MD, Daniel Moon, MD, Upper limb prosthetics. 9/20/2014
Previous Revision(s) of the Topic
Laura Gruber, MD, Tiffany M. Lau, MD. Upper Limb Prosthetics. 4/29/2021
Author Disclosure
Alberto Esquenazi, MD
Ossur, Stipend, Board member